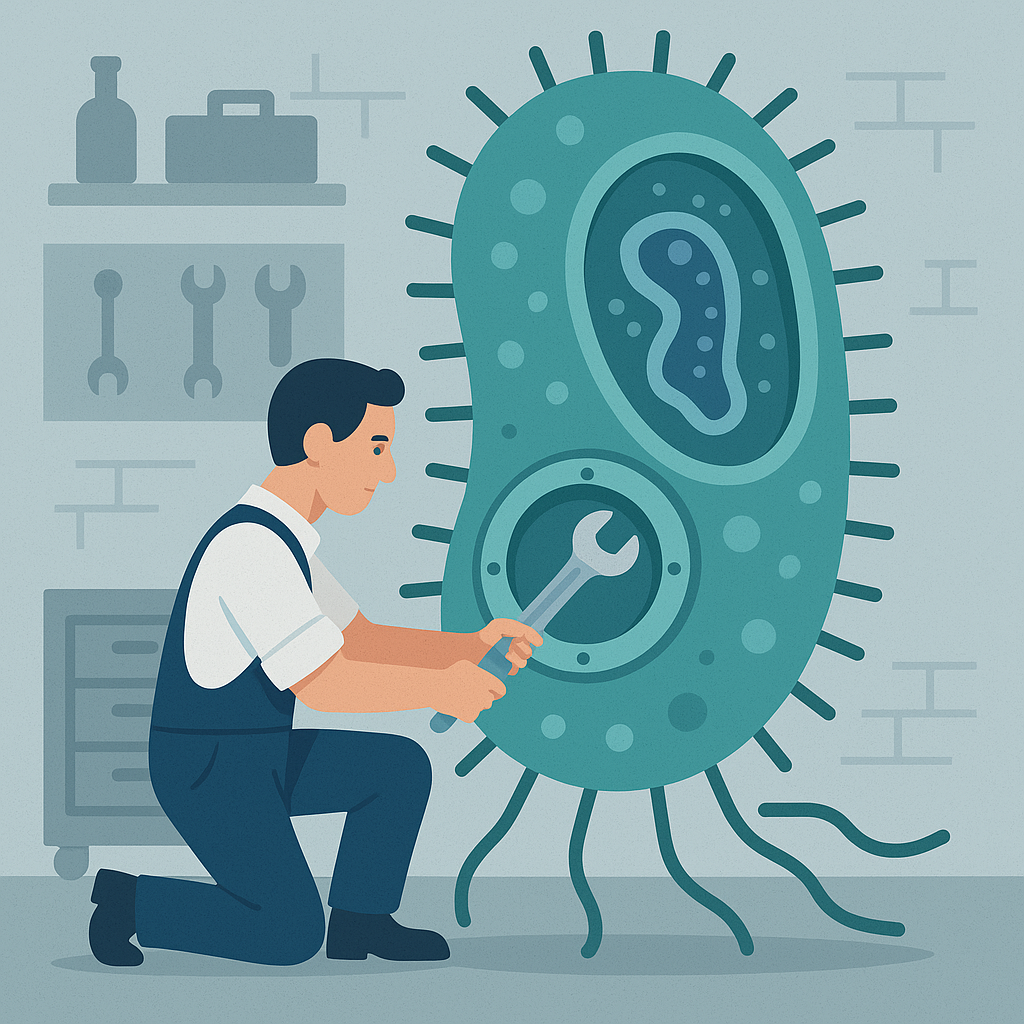
An Introduction to the Microbiome Engineering Toolkit
Share
Perhaps you’re a clinician aiming to restore balance to a patient’s disrupted gut microbiome after antibiotic treatment, or a farmer interested in enhancing crop yields by optimizing the soil microbial community. Maybe you're an environmental engineer looking to clean up contaminated groundwater using a pollutant-degrading bacterial strain. Whatever the scenario, once a clear objective is established and the microbiome in question has been adequately characterized, the next critical step is choosing the appropriate strategy and tools for achieving your desired outcome. Microbiome engineering requires thoughtful consideration of how best to steer or reconstruct these complex microbial communities, turning scientific insights into practical solutions.
Strategies for Engineering Microbial Communities
When scientists and engineers set out to “engineer” a microbiome, they generally follow one of two philosophies: top-down or bottom-up. The difference lies in whether we start with an existing complex community or build one from scratch, and it reflects the design intent – are we manipulating a whole ecosystem as it is, or assembling one piece by piece?
- Top-Down Approach: A top-down strategy treats the microbiome as a black box ecosystem that we can steer by changing external conditions or applying selective pressures. It’s like tending to an overgrown garden by pruning certain plants and adjusting the soil nutrients to encourage desired flowers to bloom. In microbiome terms, top-down engineering might involve changing a person’s diet, using drugs to inhibit certain microbial activities, or other interventions that nudge the entire community toward a healthier state. The key is that we begin with the full, complex microbiome and attempt to manipulate it from above – influencing which microbes thrive or decline by tweaking environmental variables or applying stressors. This approach is often pragmatic when dealing with systems like the human gut: rather than isolating and reintroducing every microbe, we try to create conditions that favor beneficial species already present and suppress the harmful ones.
- Bottom-Up Approach: In contrast, a bottom-up strategy is akin to building the microbiome from parts. Rather than working with the entire wild garden, imagine designing a new garden bed by carefully selecting each plant species and planting them in combination. Bottom-up microbiome engineering starts with known individual strains or a defined set of microbes and assembles them into a community with a specific goal. Scientists might cultivate a consortium of bacteria in the lab that, when introduced into a host or environment, can perform a desired function (like breaking down a pollutant or producing a needed nutrient). This bottom-up method uses prior knowledge of how certain microbes behave and interact to design a custom community. The intent is to have control over the composition from the outset, creating a microbiome that is tailor-made for a task. Bottom-up approaches benefit from precision and predictability (since you know which microbes are there), but it can be challenging to get a constructed community to successfully establish itself in a real, competitive ecosystem.
Both top-down and bottom-up microbiome engineering have their merits and challenges. Top-down methods can harness the resilience and complexity of an existing microbiome, but changes can be unpredictable and it may be hard to target specific outcomes in a soup of thousands of species. Bottom-up methods offer control by using defined microbes, yet these designer communities might struggle to compete or remain stable when faced with the incumbent microbial residents in a host or environment. In practice, scientists often see these approaches as complementary – for example, you might introduce a designed probiotic community (bottom-up) into a patient after using antibiotics to clear some of the existing microbes (a top-down move), thus making space for the newcomers. No matter the strategy, the end goal is similar: to reshape the microbiome in a way that improves health or functionality.
Whether using a top-down or bottom-up design (or a bit of both), microbiome engineers have a variety of tools and techniques at their disposal to intervene in microbial communities. These interventions can be grouped into three broad categories based on how they alter the microbiome:
- Additive approaches – adding or introducing beneficial microorganisms or genes to the community.
- Subtractive approaches – removing or killing specific undesirable microorganisms.
-
Modulatory approaches – changing the environment or conditions to influence microbial behavior and composition without directly adding or removing microbes.
Think of it this way: to fix an unbalanced microbiome, you can add something good, remove something bad, or tweak the settings to encourage a return to harmony. Let's explore each of these categories and the key modalities or tactics that fall under them.
Additive Strategies: Introducing New Function
Additive microbiome interventions are all about enriching the community with helpful microbes that were absent or depleted. If a microbiome is like a team missing some crucial players, additive approaches try to draft new team members who can restore balance or provide a missing function. These strategies introduce new microbial members to an ecosystem, whether it’s the human gut, a crop’s root microbiome, or any other microbial habitat. Here are some of the main additive tools in the microbiome engineering toolkit:
Microbial Inoculants: Microbial inoculants are perhaps the most common and familiar additive approach in microbiome engineering. These consist of carefully selected live microorganisms—whether probiotics designed for human health, plant-growth-promoting bacteria added to soil, or bioaugmentation strains introduced to remediate environmental contaminants—that confer specific benefits to their target environment. The principle behind inoculants is straightforward: by introducing beneficial microbes, the goal is to enhance or restore important microbial functions that may be diminished or absent. For instance, in agriculture, inoculants containing nitrogen-fixing bacteria can improve soil fertility and crop productivity, while in environmental remediation, bioaugmentation strains help degrade pollutants like petroleum hydrocarbons. In human health, probiotics—such as certain Lactobacillus or Bifidobacterium strains—are administered to replenish beneficial gut microbes after antibiotics disrupt the intestinal microbiome. Inoculants may involve a single microbial strain or carefully designed consortia of several complementary microbes, each selected for specific beneficial traits. While their effectiveness can vary depending on compatibility with the existing microbiome, environmental conditions, and strain selection, microbial inoculants provide a direct and practical method for enhancing microbiome function and achieving targeted microbiological outcomes.
Microbiota Transplants: Beyond just single strain inoculants or small consortia, it is also possible to transplant an entire microbial ecosystem into another system that has become highly imbalanced or dysfunctional. This concept is perhaps best exemplified by fecal microbiota transplantation (FMT) in human health, where a processed sample containing a diverse array of microbes from a healthy donor is introduced into a patient’s gastrointestinal tract. FMT has shown remarkable success, particularly in treating recurrent Clostridioides difficile infections, by restoring a balanced microbial community that can outcompete and displace harmful pathogens.
Yet, the idea of transplanting entire microbial communities extends far beyond the human gut. In livestock management, for instance, rumen microbiota transplants have been explored as a means to optimize digestion and improve feed efficiency, and potentially reduce methane emissions in cattle. Similarly, in agriculture, soil microbiota transplants are being investigated to rehabilitate degraded lands, enhance nutrient cycling, and promote plant growth. Even in aquatic environments, transplanting native microbial communities is emerging as a strategy to restore balance in ecosystems such as coral reefs and freshwater habitats. In all these cases, the core principle remains the same: using a complete, functioning microbial consortium as a therapeutic or restorative transplant. This approach represents a top-down additive strategy, where instead of introducing a single species or a small group of microbes, an entire ecosystem is introduced to reestablish a stable and beneficial microbial community.
Engineered Microbial Consortia: Scientists are creating defined mixtures of microbes in the lab as targeted therapies. Instead of relying on donor stool (which contains hundreds of unknown species), one approach is to formulate a synthetic consortium – a set of specific strains chosen because they work well together or provide complementary functions. For example, one bacterium might produce a beneficial nutrient while another consumes a harmful compound. By mixing such strains into a controlled cocktail, researchers can craft a standardized therapeutic community that can be administered like a drug. This bottom-up additive approach offers consistency and safety (the composition is known and can be screened for pathogens). The challenge, however, is ensuring these introduced microbes successfully establish themselves in the patient’s existing microbiome. Often an additive intervention like this is paired with a subtractive step (say, a mild antibiotic pretreatment) to open up space for the newcomers to take root.
Gene Delivery Strategies: Another powerful additive strategy involves the direct delivery of beneficial genes into existing microbiomes. Rather than simply introducing new microbial strains, these approaches utilize engineered organisms or genetic vehicles to transfer specific functional genes directly into resident microbes. For example, conjugative vectors, which are plasmids capable of moving genetic material between bacteria, can be used to distribute desirable traits—such as pollutant degradation or antibiotic resistance management—throughout a microbial community. Similarly, engineered microbial strains equipped with particular metabolic capabilities or therapeutic functions can be introduced into environments like the human gut or contaminated soil, where they transfer beneficial genes horizontally to native community members. Additionally, engineered bacteriophages have emerged as precise gene-delivery vehicles, capable of introducing targeted genetic modifications or therapeutic payloads into specific microbial populations. However, the need for regulatory approval is often a major barrier to gene delivery strategies. Nevertheless, these approaches represent a highly customizable and precise form of microbiome engineering, allowing targeted enrichment of microbial ecosystems with desired metabolic capabilities, therapeutic molecules, or ecological functions, thereby expanding the scope of additive microbiome interventions.
All additive strategies share a common theme: they are generally intended to fill gaps in the ecosystem – whether by reintroducing beneficial microbes that have dwindled or introducing entirely new functions to the community. However, sometimes the best way to fix a problem is not by adding something, but by removing something that shouldn’t be there. This is where the subtractive side of the toolkit comes in.
Subtractive Strategies: Removing Unwanted Microbes
Subtractive microbiome interventions aim to weed out the bad actors from a community. If harmful or disruptive microbes are akin to weeds choking a garden, subtractive techniques are the weed killers and pruning shears that selectively eliminate those unwanted elements. The goal is to make the microbiome healthier by subtraction – taking away pathogens or overgrown species so that the remaining community (or subsequent additions) can flourish. Key subtractive modalities include:
Antibiotics: The most classic way to remove microbes is to use antibiotics, drugs that kill or inhibit bacteria. But antibiotics are a blunt tool when it comes to the microbiome. A broad-spectrum antibiotic can wipe out beneficial microbes along with the harmful ones – like using a weedkiller that kills your flowers along with the weeds. This often leaves the microbiome disrupted (a state of dysbiosis) where opportunistic bugs can overgrow. To be more precise, scientists are working on narrow-spectrum antibiotics and other new antimicrobials that target specific pathogens while sparing the rest of the community. Antibiotics can certainly knock back a troublesome microbe when needed, but due to their collateral damage, there's a push to find gentler, more targeted subtractive methods.
Bacteriophages: Bacteriophages (or simply "phages") are viruses that specifically infect and kill bacteria, offering a highly precise subtractive tool for microbiome engineering. Due to their narrow host range, phages can selectively eliminate particular bacterial species or even specific strains, leaving beneficial members of a microbial community largely unaffected. While historically best known for phage therapy—treating antibiotic-resistant bacterial infections in humans—phages are also extensively used in agricultural and environmental settings as agents of biocontrol. In agriculture, for example, phages can target pathogens that cause plant diseases, reducing reliance on broad-spectrum pesticides. Similarly, in food production, phages have been deployed to selectively reduce foodborne pathogens, enhancing food safety without disrupting desirable microbiota. Beyond these direct antibacterial applications, phages are increasingly being explored as "microbiome editing" tools, capable of subtly reshaping microbial ecosystems by selectively removing or suppressing problematic bacterial populations. Although challenges remain, such as bacterial resistance and the need for tailored phage selection, their unparalleled specificity makes bacteriophages an attractive subtractive approach for targeted microbiome manipulation across diverse systems.
Bacteriocins: Bacteria often produce their own antibiotics to battle each other. These bacterium-made toxins, called bacteriocins, can kill specific unwanted microbes. In microbiome engineering, they can be harnessed as precision weapons. For example, one could deploy a harmless bacterium that secretes a bacteriocin to knock down a pathogen like Salmonella right in the gut. Because many bacteriocins have a very narrow killing range, like phages, they can serve as molecular scalpels – removing the bad actor while sparing most of the surrounding microbiome. However, their narrow spectrum of activity makes it challenging to address microbiome imbalances involving multiple or diverse pathogens. Additionally, bacteria can develop resistance to bacteriocins, potentially reducing their long-term effectiveness if not implemented properly.
Modulatory Strategies: Fine-Tuning the Microbial Environment
Modulatory approaches in microbiome engineering focus on changing the habitat or resources of the microbial community to influence its composition and activity. Think of these as indirect strategies – instead of introducing new microbes or killing off existing ones, you adjust the “settings” of the ecosystem so that the microbiome shifts on its own. It’s comparable to tending a garden by adjusting sunlight, water, or fertilizer: you’re not planting new seeds or pulling weeds one by one, but by enriching the soil or changing the climate, you encourage certain plants to grow better and others to fade. In a microbial sense, modulatory interventions might include dietary changes, specialized supplements called prebiotics, or other chemicals that alter microbial behavior. Such strategies cannot add function to a microbiome if it’s not already present, but can help enhance or reduce pre-existing functions. Here are some key modulatory tools:
Nutrient Modulation: Adjusting nutrient availability is a powerful modulatory strategy for shaping microbial community composition. By strategically adding or limiting key nutrients such as carbon, nitrogen, phosphorus, or oxygen, microbiome engineers can selectively encourage the growth of desirable microbial species while restricting the proliferation of others. For instance, in soil microbiomes, increasing nitrogen or phosphorus availability can enrich specific beneficial bacteria or fungi associated with plant growth, while deliberate nutrient limitation (like oxygen starvation or phosphate reduction) can suppress unwanted species or metabolic activities. Similarly, dietary prebiotics—specialized nutrients like complex carbohydrates and fibers—selectively promote beneficial gut bacteria in humans and animals, indirectly limiting the growth of pathogens through competitive exclusion. Across diverse environments, targeted nutrient manipulation allows engineers to shape microbial communities by creating favorable conditions for desired microbes, thus indirectly steering the microbiome toward specific functional outcomes.
Environmental Adjustments: Beyond nutrition, microbiome composition can be strongly influenced by altering physical and chemical environmental conditions. Parameters like temperature, pH, moisture, and salinity profoundly shape microbial ecosystems, determining which species thrive and which are suppressed. In practice, changing the pH of agricultural soil or fermentation processes can shift the microbiota towards desired species and functions. Similarly, temperature shifts—such as those used in industrial bioprocessing—can selectively favor thermophilic microbes with beneficial metabolic pathways. These environmental interventions rely on adjusting ecological conditions, serving as indirect modulatory methods that push existing microbial communities to reorganize naturally according to new selective pressures.
Small Molecules: Another nuanced approach involves the strategic use of small molecules to subtly influence microbial behavior without directly adding or removing microbes. These interventions can disrupt specific microbial signaling pathways such as quorum sensing—the process by which bacteria coordinate collective behaviors like biofilm formation and toxin production. By introducing small molecules that block or alter quorum sensing signals, harmful bacterial behaviors can be inhibited, tipping the balance toward a healthier or more stable microbiome without necessarily killing the targeted microbes. Additionally, other targeted small molecules might selectively inhibit microbial enzymes, alter metabolic pathways, or change microbial growth rates. Such targeted chemical interventions offer precision and specificity, allowing microbiome engineers to subtly and precisely shape microbiome function and composition.
Conclusion
Engineering a microbiome is a bit like taming a wilderness or conducting an orchestra – it requires understanding the ensemble and knowing when to add, remove, or subtly influence each part. We’ve introduced the major categories in the microbiome engineering toolkit: additive approaches to populate an ecosystem with helpful microbes, subtractive approaches to remove harmful ones, and modulatory approaches to change the environmental tune that the microbes dance to.
Real-world microbiome interventions often blend these strategies. For instance, treating a gut infection might involve a subtractive step (antibiotics or phages to clear the pathogen), followed by an additive step (probiotics or an FMT to restore diversity), and supported by modulatory guidance (dietary recommendations to maintain the restored balance). As research progresses, we’re learning how to better target these interventions – using genomic insights to pick the right probiotic strains, engineering phages for precision, and identifying which dietary components most strongly influence key microbes.
In summary, the microbiome engineering toolkit provides multiple levers – add, subtract, modulate – to tune microbial communities. It’s a toolkit that is expanding rapidly, fueled by an ever-growing understanding of the microbiome’s role in our lives. With careful application and further research, these approaches have the potential to turn microbiomes into allies in medicine, agriculture, and environmental sustainability. The field is still young, but the concept is powerful: by masterfully adding, removing, and tweaking elements of the microbial world, we can craft healthier and more resilient ecosystems both within us and around us.